Research Highlights
Articles
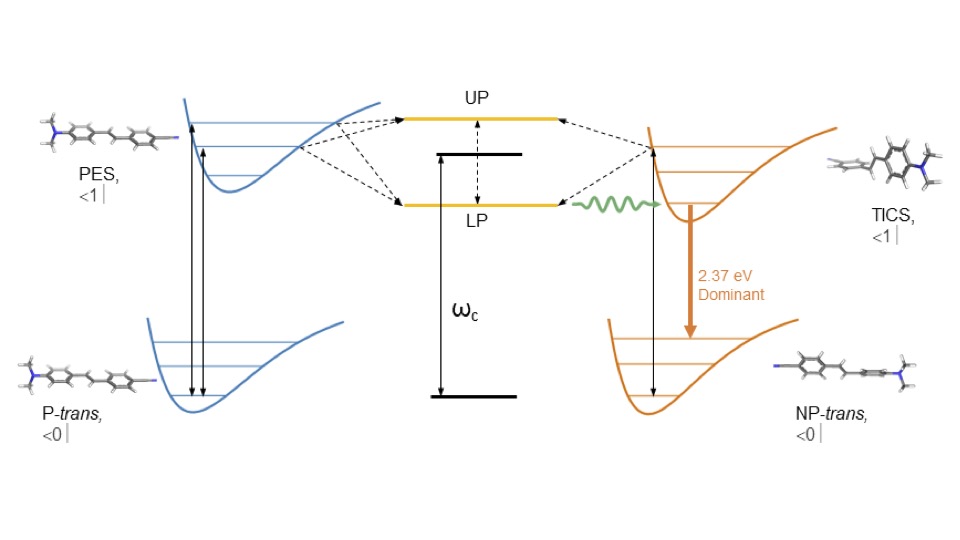
Polaritons are hybrid light-matter excitations that can form when material excitations are sufficiently strongly coupled to confined light modes in optical cavities. Polaritons in organic systems have shown the potential to modify chemical properties and to mediate long-range energy transfer between individual chromophores, among other capabilities. Thanks to an experiment-theory collaboration between the group of Prof. Vinod Menon at City College of New York and IFIMAC researchers Francisco J. Garcia-Vidal and Johannes Feist, it has been demonstrated that strong coupling and formation of organic exciton-polaritons can be also used to selectively tune the light emission of organic molecules to come from a specific isomer. Molecular isomers are formed by different arrangements of the same atoms in space. By taking advantage of the delocalized and hybrid character of polaritons, their emergence in the strong coupling regime opens a new relaxation pathway that allows for an efficient funneling of the excitation between the molecular isomers. The researchers have implemented this idea by strong coupling to trans-DCS (E-4-dimethylamino-4′cyanostilbene) molecules, which present two isomers when immersed in a polymer matrix, with the relative amount being controllable by the molecular concentration. Under normal conditions, the non-planar (NP) isomer is in the minority and does not contribute significantly to emission. Within the cavity, the new relaxation pathway opened by polariton formation allows to selectively funnel the photoexcitation from a common polaritonic mode (the lower polariton LP) to the excited states of the NP isomer, leading to pure emission from this isomer. This approach thus provides a pathway to significantly modify the emission wavelength of a molecular material, which could be useful for the design of novel light-emitting devices. [Full article]
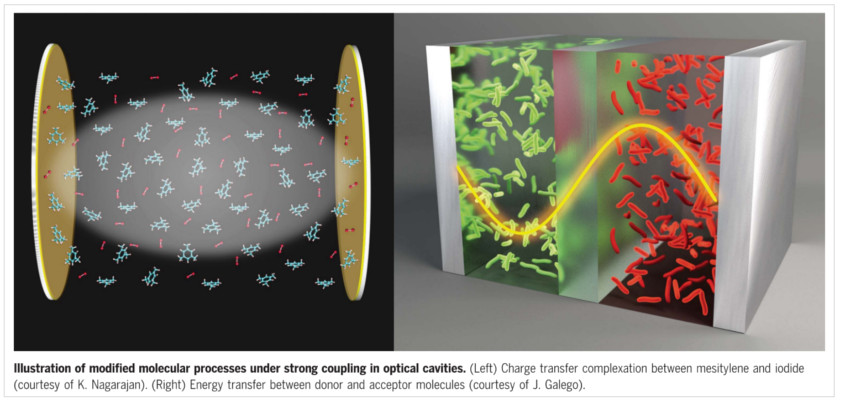
Laser technology is a familiar example of how confining light between two mirrors can tune its properties. Quantum mechanics also dictates that even without extraneous light, matter confined in a cavity resonant with its electronic or vibrational transitions can couple with vacuum electromagnetic field fluctuations. In this paper, Garcia-Vidal and co-workers review the remarkable and still somewhat mysterious implications of this “strong-coupling” regime.
Both experimental and theoretical studies have shown changes to photochemical reaction rates under strong coupling between the electronic excitations of molecules and cavity electromagnetic modes. Strong coupling modifies the shape of the potential energy surfaces associated with the excited states of the molecule, allowing for a manipulation of its photophysical properties. Moreover, ground-state chemical reactivity can also be completely modified when molecular vibrations are strongly coupled to infrared cavity modes. Although a detailed picture of the mechanism is still missing, symmetry seems to play a key role. Material properties can also be changed by strong coupling. Charge and energy transport in organic materials and magneto-conductivity in two-dimensional electron gases have been shown to be altered. Thanks to the intrinsic delocalized character of the polaritonic modes, transport properties can be then tuned at a macroscopic scale. It is also feasible to manipulate phases of matter by means of strong coupling. It has been reported that the critical temperature of a superconductor can be substantially enhanced by judiciously exploiting vibrational strong coupling and that the ferromagnetism of nanoparticles can be boosted by orders of magnitude. These examples illustrate the potential of using vacuum fields instead of intense laser fields to induce modification of material properties. [Full article]
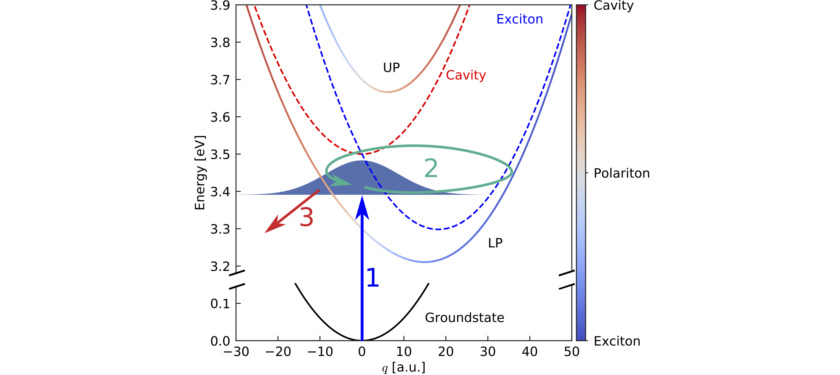
The motion of atoms in a molecule typically occurs on the femtosecond timescale (10-15 s). The standard technique used to study chemical reactions at these timescales is pump-probe spectroscopy, where first an ultrashort pump laser pulse initiates the dynamics in the molecule and, some time later, an ultrashort probe laser pulse is used to obtain information about the current state of the molecule. However, the information obtained in these approaches is always somewhat indirect. If you imagine the molecule as a musical instrument and the laser pulses as short sound pulses, these approaches correspond to monitoring the change in the sound frequencies that the instrument responds to when it has been struck by a first sound already (this is known as transient absorption spectroscopy), or even to using such a strong second pulse that it blows apart the instrument, and trying to understand its motion before falling apart by measuring the pieces that fly away. These experiments are then repeated many times with different time delays between the pulses, to construct something akin to a movie of the molecular motion after it has been excited. One important reason that these approaches are used is that simply “listening” to the molecule, i.e., measuring the light that it emits after the first pulse, normally does not provide the required information. The fundamental reason for this is that a molecule only couples quite weakly to light, which has two main consequences: First, the amount of light that is emitted is typically quite low (making it hard to “hear” the molecule), and second, it takes a long time for the light to be emitted so that even if you can measure it, it is produced at times long after the interesting motion has taken place.
A theoretical proposal by a group of researchers from the Departamento de Física Teórica de la Materia Condensada and the Condensed Matter Physics Center (IFIMAC) at the Universidad Autónoma de Madrid now provides a way to resolve both of these problems at the same time and make it possible to listen to the molecule directly in those first few crucial tens of femtoseconds. The basic idea of this work is to place a molecule in a nanoplasmonic cavity, where the strong coupling between the molecule and the electromagnetic modes of the cavity transforms the molecule into a “loudspeaker”. The nanoplasmonic cavity in this case acts both as a resonator that changes the “sound” of the molecule and as an amplifier that makes it possible to “hear” the molecule. In the article, the authors show that ultrafast molecular dynamics can be traced with femtosecond resolution by looking at the photon emission by the cavity, which only happens when the molecular vibrational wavepacket is close to a position where the molecule and the cavity are in resonance with each other. In this way, the setup acts like a clock that measures how long the molecule takes to reach a given position. In particular, the authors show that this idea works in the regime of strong light-matter coupling, where the molecule and the cavity interact so strongly that their excitations become hybrid light-matter states known as polaritons. In this regime, this novel technique could provide direct insight into the modification of molecular motion induced by polariton formation. This approach could open the window for experimental and theoretical advances in the way we explore ultrafast chemical dynamics, paving a new way to combine femtochemistry and molecular polaritonics using plasmonic nanocavities. [Full article]
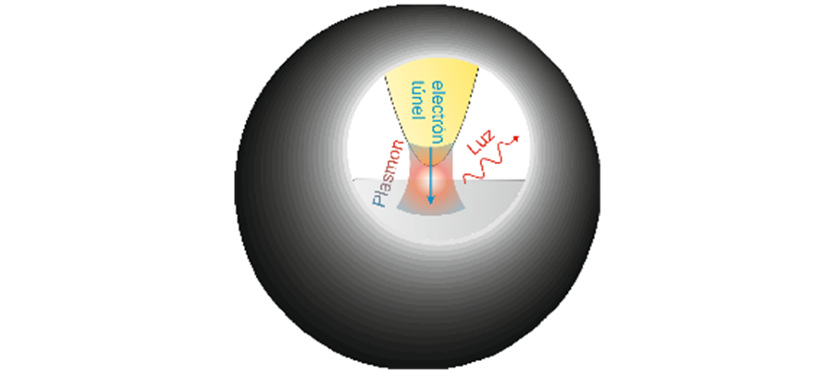
Researchers from the Universidad Autónoma de Madrid, IMDEA Nanociencia and IFIMAC have developed a new method for the fabrication and characterization of atomic-sized photonic cavities, by exploiting the mechano-quantum tunnel effect. This discovery may be fundamental for the understanding and design of new, nanometric size, opto-electronic devices which will be key for the development of new technologies based on quantum properties, such as sensors or quantum computers.
Photonic cavities are an essential part of many modern optical devices, from a laser pointer to a microwave oven. Just as we can store water in a tank and create standing waves on the surface of the water, we can confine light in a photonic resonator whose walls are strongly reflective. Just as water surface waves depend on the geometry of the tank (shape, depth), specific optical modes can be created in a photonic cavity whose properties (color and spatial distribution of intensity) can be tuned by changing the dimensions of the cavity. When the size of the cavity is very small, much smaller than the wavelength of the light confining it (nano-cavity in the case of visible light), an intensification effect of the light is produced that is so strong that it influences the electrons on the walls of the cavity. A mixture between photons and electrons is then produced, giving rise to hybrid modes between light and matter known as plasmons. Plasmons in optical nano-cavities are extremely important for many applications such as chemical sensors that allow the detection of individual molecules or the manufacture of nanolasers that could operate with hardly any electrical current consumption. However, the characterization of these plasmonic modes is generally very complex, because the tiny size of the cavities makes it extremely difficult to access them by external signals.
On the other hand, the tunnel effect is one of the most characteristic, mysterious and best documented effects of Quantum Mechanics. In a tunnel process, a particle (e.g. an electron) can pass through a narrow barrier (the space that separates two metals at nanometric distances) despite not having enough energy to overcome it. It is as if we could pass from one side to the other of the Great Wall of China without having to jump over it. Incredible as it may seem, particles from the quantum world can do this under certain conditions. In most of these processes, the energy of the particle before and after the process is the same. However, in a small fraction of these events, the particle can give up some of its energy, for example, by generating light, which is known as the inelastic tunnel process. Although it is well known that the properties of the light emitted in the inelastic tunnel process between two metals depend on the plasmonic modes that exist in the cavity, it also depends strongly on the energy distribution of the particles performing the tunnel process. Until now, it had been impossible to distinguish unequivocally between these two effects and therefore extract the information on the plasmonic modes from the analysis of the light emitted by the tunnel effect.
In an article published this week in the prestigious journal Nature Communications, researchers from the Universidad Autónoma de Madrid, IMDEA Nanociencia and IFIMAC have developed a method to solve this problem by simultaneously determining the energy distribution of the tunneling electrons and the light emitted in a Scanning Tunnel Microscope. This effort has allowed them to exploit the tunneling effect to create optical resonators of atomic dimensions and to study their optical properties, unravelling for the first time the contributions due to the energy of the tunneling particles from the effects originated by the plasmonic modes in the cavity. This work proposes a novel methodology for the characterization of light-matter interaction at atomic size and may have important technological implications for the development of chemical sensors of single molecules, new sources of single or interlaced photons or nanolasers that are active at extremely low pumping powers. [Full article]
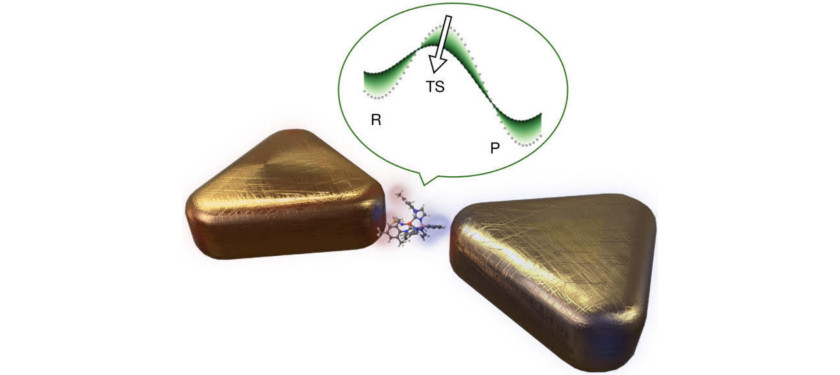
Over the past few years it has become clear that strong electromagnetic coupling between a molecule and light confined in a nanoscale cavity can lead to significant modifications of the electromagnetic response of the hybrid system as well as the internal molecular properties. This has already been exploited to manipulate the fate of photochemical reactions, however, up to now there has been no general theory on how these interactions affect the chemical reactivity of a molecule in its ground state without any external input of energy.
In a theoretical study published in Physical Review X, a group of researchers from the Departamento de Física Teórica de la Materia Condensada and the Condensed Matter Physics Center (IFIMAC) at the Universidad Autónoma de Madrid have developed a theoretical framework that combines quantum electrodynamics and the quantum theory of chemical reactivity. The authors implemented this approach for a simple model molecule that can be solved without approximations. This allowed to explore the general properties of cavity-induced ground-state chemical reactivity changes and develop a simplified theoretical model that can be applied to more complex molecules. They found that the induced changes on the molecular pontential energy surfaces do not depend on any resonance condition between molecular transitions (such as vibrational or electronic excitations) and cavity modes, with the relevant interactions closely related to well-known electrostatic forces on the molecule due to other molecules or larger material bodies. In particular, the largest contribution to the modification of the energy landscape is typically determined by the change of the permanent dipole moment of the molecule between its equilibrium and transition state configuration. They have also shown that experimentally available nanocavities can induce changes in reaction rates by an order of magnitude in a single molecule, while for the case of many molecules, this effect becomes significant only if all the molecules are aligned.
In a second paper published in Angewandte Chemie, the same authors have combined this theory with quantum chemistry calculations and have shown how plasmonic nanocavities can enable self-induced electrostatic catalysis of typical organic reactions, without any external driving, due to the interaction of the molecular permanent and fluctuating dipole moments with the plasmonic cavity modes. They have also exploited this interaction between molecules and electromagnetic modes to predict cavity-induced changes in the transition temperature of spin-crossover transition metal complexes, the prime example of molecular switches.
These studies contribute to the fundamental understanding of how nanoscale cavities can be used to manipulate chemical reactions of single molecules and open the path towards ground-state catalysis with plasmonic nanocavities. They also provide an example on how the interaction with plasmonic modes may be ultimately exploited to manipulate material properties. [Angewandte Chemie – full article][Physical Review X – full article]
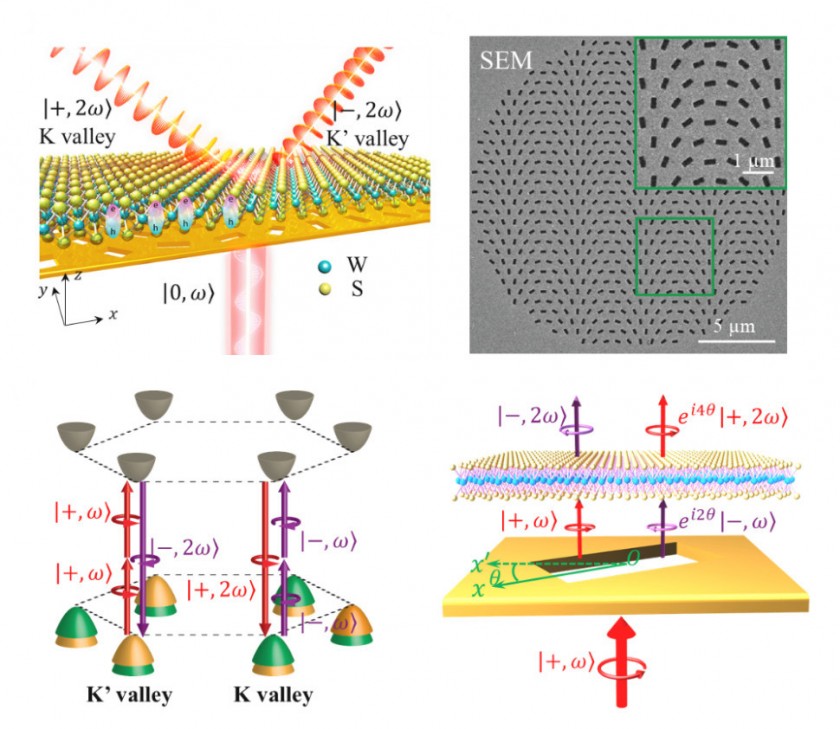
Two-dimensional transition metal dichalcogenides (TMDCs) present extraordinary nonlinearities and direct bandgaps at the K and K′ valleys. These valleys can be optically manipulated through, for example, plasmon–valley-exciton coupling with spin dependent photoluminescence. However, the weak coherence between the pumping and emission makes exploring nonlinear valleytronic devices based on TMDCs challenging. In a collaboration between IFIMAC member Francisco J. Garcia-Vidal and two experimental groups based in Singapore and China, it has been demonstrated that a metasurface (a gold film drilled with rectangular nanoholes arranged in a hexagonal lattice but with different local rotation angles), which entangles the phase and spin of light, can simultaneously enhance and manipulate nonlinear valley-locked chiral emission in monolayer tungsten disulfide at room temperature. The second-harmonic valley photons, accessed and coherently pumped by light, acquire a spin related geometric phase provided by the gold metasurface and are separated and routed to predetermined directions in free space. In addition, the nonlinear photons with the same spin as the incident light are steered owing to the critical spin–valley locked nonlinear selection rule of monolayer tungsten disulfide in the designed metasurface. This work opens a new avenue to utilize plasmonic metalsurfaces in order to build-up advanced room-temperature and free-space nonlinear, quantum and valleytronic nanodevices. [Full article]
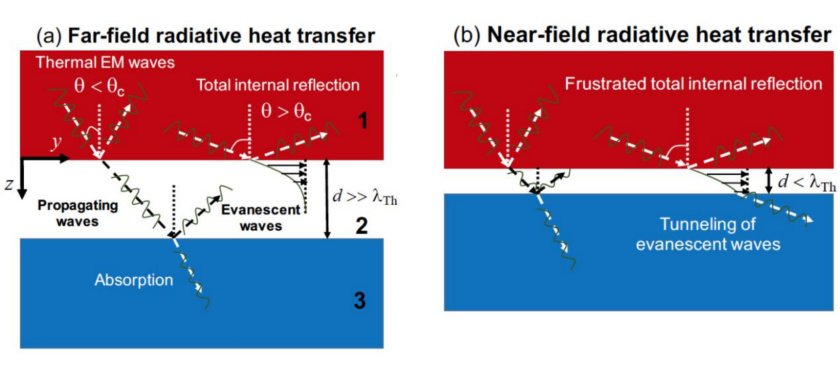
Thermal radiation is one of the most ubiquitous physical phenomena, and its study has played a key role in the history of modern physics. The understanding of this subject has been traditionally based on Planck’s law, which in particular sets limits on the amount of thermal radiation that can be emitted or exchanged. However, recent advances in the field of radiative heat transfer have defied these limits, and a plethora of novel thermal phenomena have been discovered that in turn hold the promise to have an impact in technologies that make use of thermal radiation. Now, in an article published by ACS Photonics, the IFIMAC researchers Juan Carlos Cuevas and Francisco J. García-Vidal review the rapidly growing field of radiative heat transfer, paying special attention to the remaining challenges and identifying future research directions. In particular, they focus on the recent work on near-field radiative heat transfer, including (i) experimental advances, (ii) theoretical proposals to tune, actively control, and manage near-field thermal radiation, and (iii) potential applications. They also review the recent progress in the control of thermal emission of an object, with special emphasis in its implications for energy applications, and in the comprehension of far-field radiative heat transfer. Heat is becoming the new light, and its understanding is opening many new research lines with great potential for applications. [Full article]
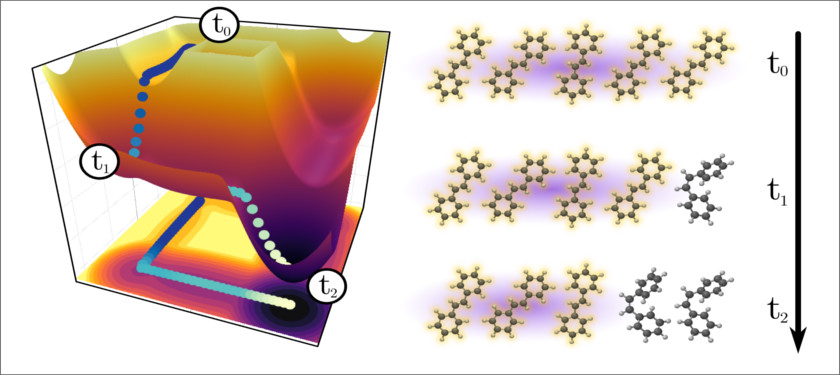
Photoisomerization is a chemical process in which the nuclear structure of an organic molecule is modified after absorption of one photon. It is of great importance to many fundamental processes in nature such as photosynthesis and human vision, and constitutes a great tool for technological applications such as optical switches and solar energy storage. In conventional photochemistry the behaviour of these reactions is ruled by the Stark-Einstein law: only one molecule undergoes a reaction per absorbed photon.
In a recent study published In Physical Review Letters, a theory group from Department of Theoretical Condensed Matter Physics and the Condensed Matter Physics Center (IFIMAC) at the Universidad Autónoma de Madrid has proven the possibility of overcoming the Stark-Einstein law in specific situations by taking advantage of the quantum electrodynamic phenomenon of light-matter strong coupling. This realization could have important applications in solar energy storage, where molecules under normal conditions efficiently absorb solar energy and store it for long times. Strong coupling could allow the release of the stored energy of the whole system on demand by using only one single photon.
In the strong light-matter coupling regime the characteristics of each component are mixed and the system develops new states called polaritons, which can show modifications in both the material and chemical properties of the system. In this work the researchers show the possibility of taking advantage of this new “polaritonic chemistry” to open new reaction pathways otherwise forbidden in conventional chemistry. They consider organic molecules proposed for solar energy storage purposes, which have a 50% probability of inducing a useful reaction when absorbing a photon. They show that by putting these molecules in the strong coupling regime, it is possible to entangle the degrees of freedom that describe the reaction of each individual molecule. This way, absorbing a photon causes one molecule to react after another, inducing a stepwise chain reaction for many molecules. Therefore, by using strong coupling it is possible to trigger a reaction of thousands of molecules using only one photon.
This work is another example of the great potential of polaritonic chemistry, of great interest for both fundamental and applied reasons. In particular it is noteworthy how this developing field brings together chemistry and quantum electrodynamics. While in standard chemical reactions light and molecules are separated and have distinct roles, polaritonic chemistry calls for a redefinition of the molecule, and enables novel and exotic chemical processes, even finding ways to defy the well-established laws of conventional photochemistry. [Full article]
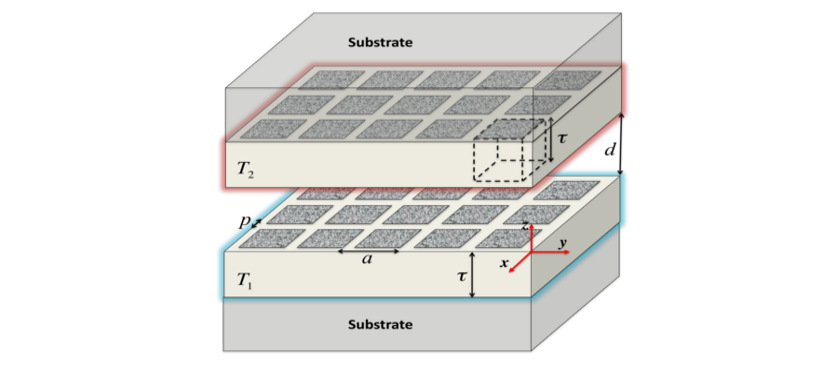
Thermal radiation is a universal physical phenomenon of great importance for different disciplines of science and engineering. In recent years, there has been a renewed interest in this topic due to the discovery that radiative heat transfer between two bodies can be drastically enhanced if they are brought sufficiently close to each other. This enhancement, which occurs when the separation is smaller than the thermal wavelength (10 microns at room temperature), is due to the contribution of evanescent waves that dominate the near-field regime. The fact that this near-field radiative heat transfer (NFRHT) between closely spaced bodies can overcome the far-field limit set by the Stefan-Boltzmann law for black bodies has now been verified in a variety of experiments exploring different materials, geometrical shapes, and gaps ranging from micrometers to a few nanometers.
In this context, the question on the fundamental limits of thermal emission is attracting a lot of attention. So far, the largest NFRHT enhancements have been reported for polar dielectrics (SiC, SiO2, SiN, etc), in which thermal radiation is dominated by surface phonon polaritons. Now, in a work published in Physical Review Letters, the IFIMAC researchers Víctor Fernández Hurtado, Francisco J. García Vidal and Juan Carlos Cuevas, together with Professor Shanhui Fan (Stanford University), have shown that metasurfaces of doped silicon can be used to boost NFRHT. In particular, they demonstrate that one can design silicon metasurfaces that not only exhibit a room-temperature NFRHT much larger than that of bulk Si or other proposed periodic structures, but they also outperform the best unstructured polar dielectric. The underlying physical mechanisms responsible for this striking behavior are the existence of broadband spoof surface-plasmon polaritons (SPPs) in doped silicion and the ability to tune via nanostructuration the dispersion relation of these SPPs that dominate NFRHT in this structure. This work illustrates the great potential of metasurfaces for the field of radiative heat transfer. [Full article]
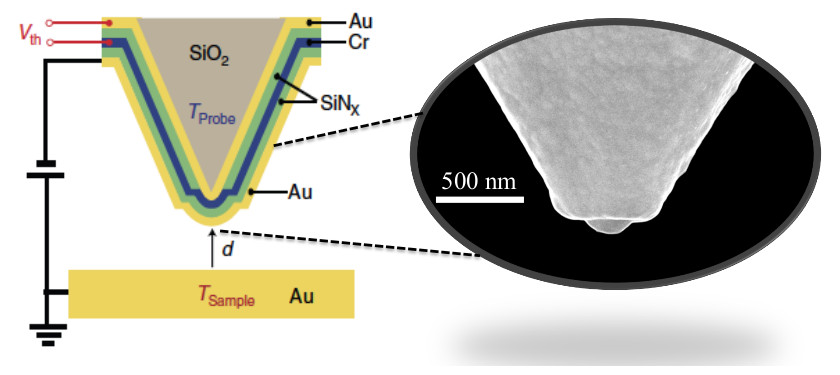
Radiative heat transfer between closely placed objects is attracting a lot attention for several reasons. First, recent experiments have finally verified the long-standing prediction that radiative heat transfer can be greatly enhanced over the classical far-field limit set by the Stefan-Boltzmann law for blackbodies if the gap between two objects is smaller than the thermal wavelength, which is of the order of 10 µm at room temperature. This is possible due to the contribution of the near field in the form of evanescent waves (or photon tunneling). Second, this confirmation has triggered the hope that near-field radiative heat transfer could have an impact in different technologies that make use of thermal radiation such as thermophotovoltaics, thermal management, lithography, data storage, and thermal microscopy.
In spite of the progress made in recent years in the understanding of thermal radiation at the nanoscale, several recent experiments exploring the radiative thermal transport in nanometric gaps have seriously questioned this understanding. In particular, measurements on two gold-coated surfaces with gap sizes in the range of 0.2-10 nm have suggested an extraordinarily large near-field enhancement more than 3 orders of magnitude larger than the predictions of fluctuational electrodynamics, which is presently the standard theory used for the description of near-field thermal radiation.
A possible solution to this puzzle has now been proposed in a work published in Nature Communications by a collaboration between the groups of Pramod Reddy and Edgar Meyhofer (University of Michigan) and IFIMAC researchers Víctor Fernández-Hurtado, Johannes Feist, Francisco J. García-Vidal and Juan Carlos Cuevas. In this work, the authors explore the radiative heat transfer in Ångström- and nanometer-sized gaps between an Au-coated scanning thermal microscopy probe and a planar Au substrate in an ultrahigh vacuum environment. Using the apparent tunneling barrier height as a measure of cleanliness, it was found that upon systematically cleaning via plasma-cleaning or locally pushing the tip into the substrate by a few nanometers, the observed radiative conductances decreased from unexpectedly large values to extremely small ones-below the detection limit of the probe-as expected from computational results obtained within the framework of fluctuational electrodynamics. These results suggest that the huge signal reported in recent experiments might be an artifact due to the presence of contaminants bridging the gap between the tip and the substrate, thus providing an additional path for heat transfer via conduction. Moreover, this work shows that it is possible to avoid the confounding effects of surface contamination and systematically study thermal radiation in Ångström- and nanometer-sized gaps. [Full article]
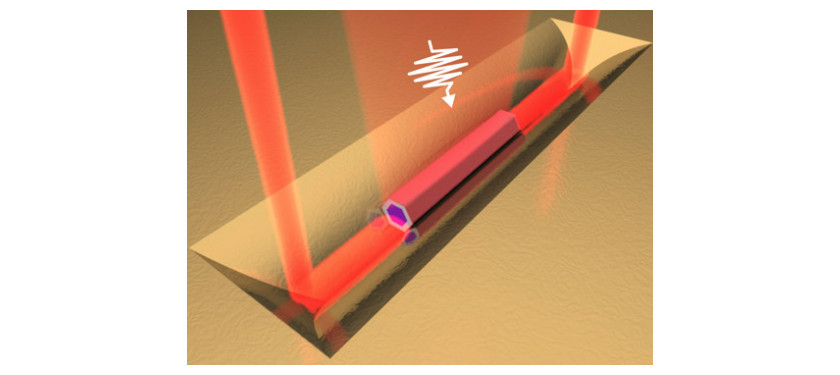
The need of ever faster and powerful data processing and communication technologies, as well as for ultrasensitive and compact sensors is playing a crucial role in the advance of novel on-chip optical devices. It is a matter of time before integrated photonic circuits will become inherent in our daily lives in a similar way that electronic circuits did with the development of the transistor. Currently, one of the main goals in nano-photonics research is being able to integrate all the key necessary building blocks on a same chip, including the light source, transmission lines, modulators and detectors. Small on-chip lasers have emerged over the last decade as an ideal solution for the light source integration, however the main challenge is to efficiently couple their emission into the small footprint components.
Now, in a recent Nanoletters publication, a group of researchers from the Departamento de Física Teórica de la Materia Condensada and the Condensed Matter Physics Center (IFIMAC) at the Universidad Autónoma de Madrid, together with researchers from the Institute of Photonic Sciences (ICFO), the Laboratory for Semiconductor Materials at the EPFL in Switzerland, the Technical University of Denmark and the Center for Nano Optics in Denmark, report on the realization of a novel ultracompact hybrid nanolaser source operating at room temperature consisting of semiconductor nanowire (NW) lasers directly integrated with wafer-scale lithographically designed plasmonic waveguides.
To achieve this, the researchers used epitaxially grown GaAs NWs with diameters at least ten times smaller than a human hair and a few microns in length, which provided both the gain medium and the cavity geometry required for the lasing emission. The NWs were then transferred from their host substrate onto a silicon chip containing gold V-groove (VG) plasmonic waveguides, which support a special type of surface plasmons with sub-wavelength lateral electromagnetic field confinements and good propagation characteristics, namely the channel plasmon polaritons (CPPs). Later, by means of micro and nanomanipulation techniques, the researchers deterministically positioned individual NWs at the bottom of the VG channels, forming the so-called NW-VG hybrid nanolaser.
Lasing action and emission coupling to the plasmon CPP waveguide mode was observed upon pulsed illumination of the NW. Furthermore, rigorous theoretical simulations revealed that the lasing action in these devices was enabled by a hybrid photonic-plasmonic mode supported by the NW-VG geometry. The demonstrated system exhibited an unprecedented transfer efficiency of the lasing emission into the CPP waveguide mode of nearly 10%. This benchmark result was possible thanks to the good overlap between the hybrid plasmon lasing mode and the subwavelength confined CPP mode of the waveguide.
The authors envision that the unique features of the developed integrated source will provide the means to build high-sensitivity chemical or bio-sensing platforms powered by an integrated nanolaser. Future directions will also focus on the development of electrical injection as well as the integration of other on-chip components along the VG to build functional photonic circuitry, for example, in quantum optics experiments employing single quantum emitters coupled to the CPPs or integrating electro-optic elements in high-speed plasmonic modulators for data communications, which overall can bring us one step closer to future hybrid photonic-plasmonic circuit platforms. [Full article]
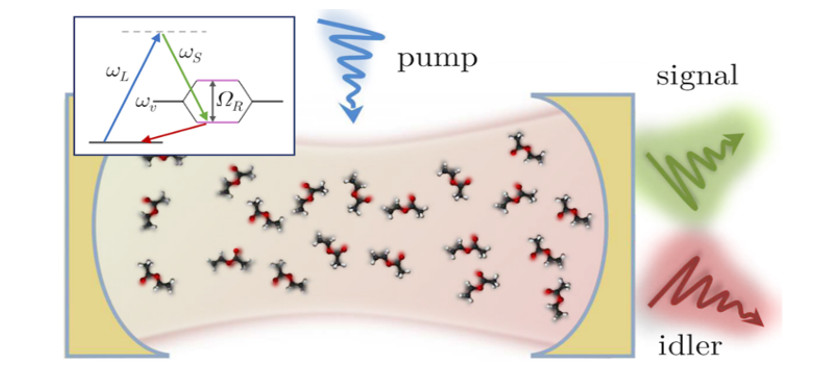
Raman scattering is a nonlinear optical process in which a photon is converted into two new excitations: a photon of a different color, as well as a material excitation such as a phonon (a quantum of vibrational energy). In a Raman laser, stimulated Raman scattering is exploited to create sources of coherent light with a wide range of achievable output wavelengths. This concept can be implemented in a variety of configurations, such as under pulsed or continuous operation, and using a wide range of nonlinear media such as optical fibers, nonlinear crystals, gases, or semiconductor materials. However, all these devices share the same drawback: Only the photon resulting from Raman scattering is used, while the material excitation is “lost” and its energy deposited in the form of heat.
In a theoretical study published in Physical Review Letters, a group of researchers from the Departamento de Física Teórica de la Materia Condensada and the Condensed Matter Physics Center (IFIMAC) at the Universidad Autónoma de Madrid present a proposal to circumvent this drawback of conventional Raman lasers. The crucial step is to modify the vibrational excitations in the material, converting them into hybrid light-matter excitations that have a photonic component, so-called vibropolaritons. This can be achieved through so-called “strong coupling”, which occurs when the coupling between the vibrational excitations and a resonant confined light mode becomes faster than the decay of either constituent. The resulting vibropolaritons then decay most efficiently through emission of light, which has two important consequences in the context of Raman lasers: First, the heat deposition is strongly reduced, as this energy is now emitted as photons. Second, and even more importantly, the Raman laser with only a single output beam is converted into a different type of nonlinear optical device, a so-called optical parametric oscillator with two output beams. These beams are coherent and have a stable phase relation, as well as providing possibly entangled pairs of photons with non-classical correlations.
In addition, the authors show that the coexistence of two vibropolariton modes with similar properties allows to operate the same device as an all-optical switch. Here, one (gate) pump beam can be used to switch on or off the Raman lasing of a second (signal) pump beam with slightly detuned frequency. In this mode of operation, both vibropolariton modes show stimulated emission as soon as the total pump power becomes large enough, such that the device produces three coherent output beams.
These results are an example of the great potential that hybrid light-matter states possess in manipulating light fields and providing novel light sources. The proposed setup could be used in novel solid-state microcavity devices for applications requiring mutually coherent and/or entangled beams in disparate frequency regions, such as quantum information transmission and storage. In addition, this approach could improve existing Raman lasers by lowering the operating threshold and reducing heat generation. [Full article]
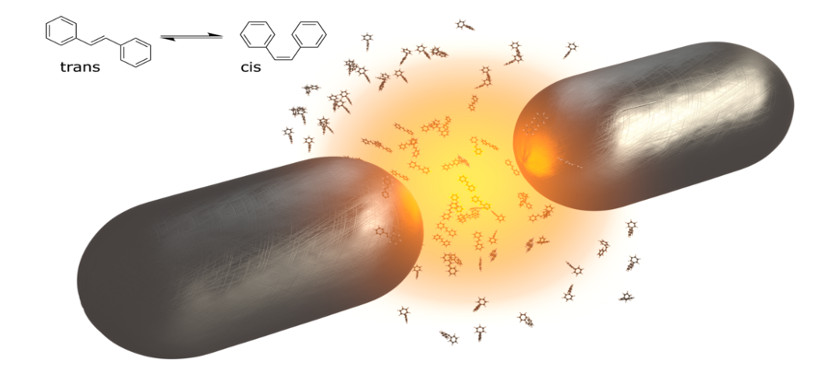
Photoisomerization is a change in chemical structure of a molecule after the absorption of a photon. It plays a crucial role in biological processes, for example in human vision, as well as for technological applications such as solar energy storage, optical memories, or molecular switches. However, photoisomerization can also have detrimental effects, for example as an important damage pathway of DNA, or as a limiting factor for the efficiency of organic solar cells. While photoisomerization can be avoided by shielding the system from light, this is of course not a viable approach for devices that rely on the interaction with external light (such as solar cells).
In a theoretical study published in Nature Communications, a group of researchers from the Departamento de Física Teórica de la Materia Condensada and the Condensed Matter Physics Center (IFIMAC) at the Universidad Autónoma de Madrid show how it is possible to take advantage of the phenomenon of strong light-matter coupling to significantly suppress photoisomerization and similar photochemical reactions.
Strong light-matter coupling is a well-known concept in quantum electrodynamics that has received a lot of attention in recent years. A quantum emitter (for example an organic molecule) and a light mode (a confined photon) become strongly coupled when the molecule can repeatedly emit a photon into the confined mode and reabsorb it before it is lost to the environment. The natural states of the system then become new hybrid states with mixed light-matter properties, known as polaritons. Strong coupling is chiefly achieved by exploiting the tools of nanophotonics and nanoplasmonics, which allow to create and design structures that can confine light to small spaces of only a few nanometers, such as in the gap between two metallic nanoparticles as sketched in the figure.
The researchers show that strong coupling in the molecule-photon system leads to a change in the energy landscape of the reacting molecule, creating an energy barrier that blocks the natural pathway from one isomer to the other. Under irradiation with an external light source (such as sunlight or a laser), the excited light-molecule state (polariton) then is significantly more stable than a molecule by itself.
Going beyond the single-molecule case, the researchers next studied the case of a large group of molecules coupled to a single electromagnetic mode. In this situation, the polariton is formed by distributing the excitation coherently over all of the molecules, enhancing the effective light-matter coupling. This is the most common way of achieving strong coupling experimentally. However, previous studies had found that the collective enhancement does not apply for changes to the internal structure, such that one could expect that the stabilization of the molecules would disappear in this setting. In a surprising twist, the opposite turns out to be the case: The researchers found that the stabilization effect becomes even more pronounced for a large number of molecules. The reason for this lies exactly in the collective nature of the excitation: Because it is distributed over many molecules, each molecule spends most of the time in its chemically stable ground state, which does not undergo isomerization. This leads to an additional collective protection of the polariton when many molecules are involved.
The study thus shows that thanks to their hybrid light-matter nature, polariton modes can be significantly more chemically stable than excitations in bare molecule, and even more so in the common experimental case of collective strong coupling. This new kind of collective protection could pave the way towards novel polaritonic materials for devices such as solar cells. [Full article]
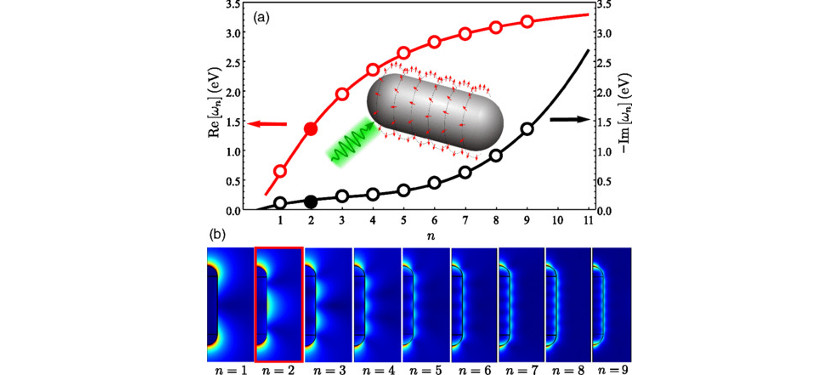
The phenomenon of collective strong coupling emerges when an ensemble of quantum emitters interacts strongly enough with an electromagnetic field. In this regime, hybrid light-matter states, the so-called polaritons, are created. These hybrid modes have the most advantageous properties of their light and matter components and this is why they are usually considered as feasible ingredients in future quantum technologies. Apart from these polaritons, within the Hilbert space there are also many dark states whose origin stems from excitations within the quantum emitters that are not coupled to the light field. Up to date, these modes have been dismissed as it is thought they do not exhibit the promising properties of polaritons.
In this work we have demonstrated that this is not the case and that dark states can indeed inherit the delocalized character of the polaritons. We show that these unexpected properties only appear when the electromagnetic field displays a discrete spectrum. When the photonic structure is extended and its electromagnetic spectrum is continuous, the customary picture described above of dark states being localized is fully recovered. We also demonstrate, both numerically and analytically, that dark states can even have superior delocalized properties than polaritons when the main loss mechanism in the system is associated with the light field. [Full article]
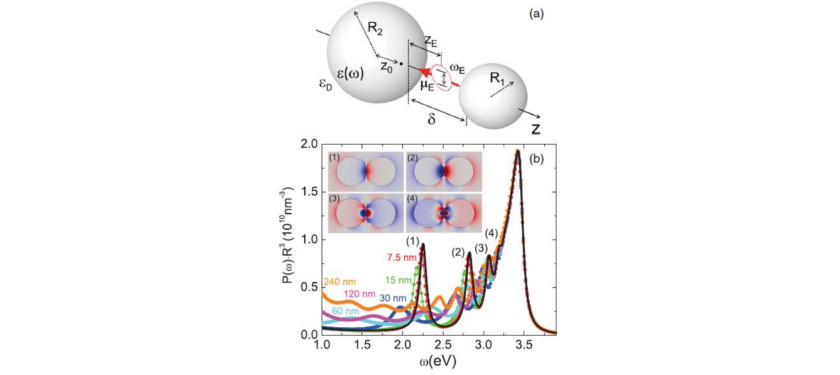
We investigate the conditions yielding plasmon-exciton strong coupling at the single emitter level in the gap between two metal nanoparticles. Inspired by transformation optics ideas, a quasianalytical approach is developed that makes possible a thorough exploration of this hybrid system incorporating the full richness of its plasmonic spectrum. This allows us to reveal that by placing the emitter away from the cavity center, its coupling to multipolar dark modes of both even and odd parity increases remarkably. This way, reversible dynamics in the population of the quantum emitter takes place in feasible implementations of this archetypal nanocavity. [Full article]
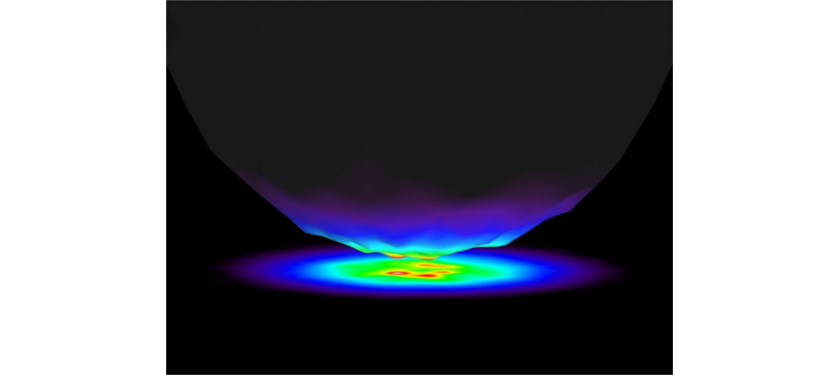
Radiative heat transfer between objects at different temperatures is of fundamental importance in applications such as energy conversion, thermal management, lithography, data storage, and thermal microscopy. It was predicted long ago that when the separation between objects is smaller than the thermal wavelength, which is of the order of 10 µm at room temperature, the radiative heat transfer could be greatly enhanced over the theoretical limit set by the Stefan-Boltzmann law for blackbodies. This is possible due to the contribution of the near field in the form of evanescent waves (or photon tunneling). In recent years, different experimental studies have confirmed this long-standing theoretical prediction. However, and in spite of this progress, recent experiments exploring the radiative thermal transport in nanometric gaps have seriously questioned our present understanding of thermal radiation at the nanoscale. In particular, these experiments cast some doubt on the validity of fluctuational electrodynamics, which is presently the standard theory for the description of near-field radiative heat transfer (NFRHT).
This fundamental puzzle has now been resolved in a work published in Nature by a collaboration between the groups of Pramod Reddy and Edgar Meyhofer (University of Michigan), the IFIMAC researchers Víctor Fernández-Hurtado, Johannes Feist, Francisco J. García-Vidal and Juan Carlos Cuevas, and Homer Reid (Massachusetts Institute of Technology). In this work, the authors used a novel type of scanning thermal probes with embedded thermocouples to measure the NFRHT between different materials (dielectrics and metals) down to gaps as small as 2 nm. In particular, it was shown how heat transfer between silica-silica, silicon nitride-silicon nitride and gold-gold surfaces exhibits a dramatic enhancement as the gap is reduced down to a few nanometers. Moreover, state-of-the-art simulations using the framework of fluctuational electrodynamics were able to reproduce all the experimental observations without any adjustable parameters. These results unambiguously demonstrate that fluctuational electrodynamics based on Maxwell’s equations provides an accurate description of the NFRHT between both metals and dielectrics all the way down to nanometer-size gaps. This work clarifies the fundamental mechanisms that govern the radiative heat transfer at the nanoscale and establishes a firm basis for the future design of novel technologies that make use of nanoscale radiative heat transfer. [Full article]
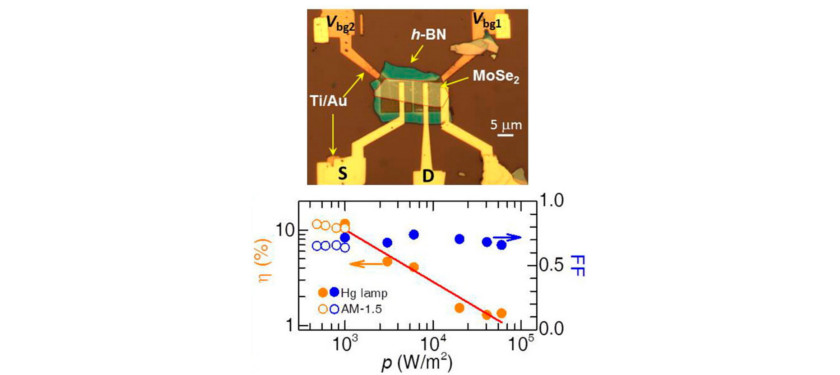
Transition metal dichalcogenides (TMDs) are layered semiconductors with indirect band gaps comparable to Si. These compounds can be grown in large area, while their gap(s) can be tuned by changing their chemical composition or by applying a gate voltage. The experimental evidence collected so far points toward a strong interaction with light, which contrasts with the small photovoltaic efficiencies η ≤ 1% extracted from bulk crystals or exfoliated monolayers. Here, we evaluate the potential of these compounds by studying the photovoltaic response of electrostatically generated PN-junctions composed of approximately 10 atomic layers of MoSe2 stacked onto the dielectric h-BN. In addition to ideal diode-like response, we find that these junctions can yield, under AM-1.5 illumination, photovoltaic efficiencies η exceeding 14%, with fill factors of ∼70%. Given the available strategies for increasing η such as gap tuning, improving the quality of the electrical contacts, or the fabrication of tandem cells, our study suggests a remarkable potential for photovoltaic applications based on TMDs. [Full article]
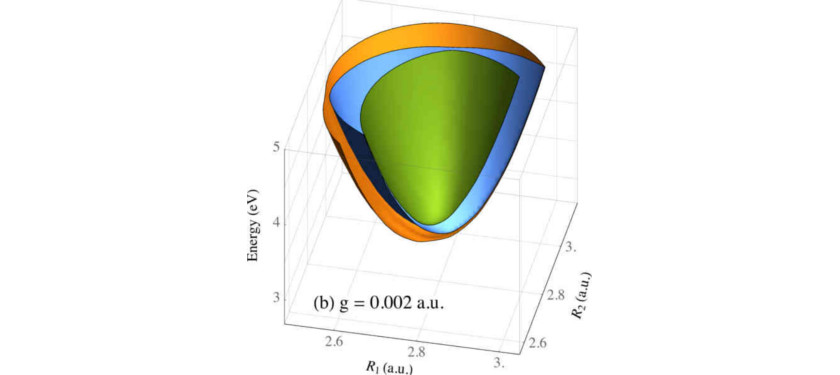
When organic molecules interact with light modes confined in nanostructures, hybrid light-matter states (polaritons) can be created via so-called “strong coupling.” The participating molecules are affected by the interaction, and this effect can be used for controlling chemical reactions or modifying a material’s properties. Up until now, there has been no consensus on how these modifications occur, and there has accordingly been no way to accurately predict these modifications or design novel structures to exploit them. Now, IFIMAC researchers Javier Galego, Prof. Francisco J. García Vidal, and Johannes Feist demonstrated that it is possible to understand and predict these modifications by adapting well-known techniques from chemical physics. The extent of the modifications turns out to depend sensitively on which observable is interrogated.
Progress up until now had been hindered by the fact that organic molecules possess a large number of internal (rovibrational) degrees of freedom and do not behave like simple two-level emitters. The researchers employed a first-principles approach that takes into account electronic, nuclear, and electromagnetic degrees of freedom on an equal footing. They demonstrated that the Born-Oppenheimer approximation, which underlies most of our understanding of chemical structure and dynamics and assumes that nuclear and electronic motion can be separated, can break down under strong coupling. However, exactly how this happens can be predicted, and in addition, in many important cases it does not break down. By exploiting this approach, the researchers show that molecular structure is modified even for so-called dark states, which are typically thought to be uncoupled from electromagnetic modes. These findings provide the basis for understanding how to manipulate chemical structure and reactions through strong coupling, and could have wide-ranging implications for the design of novel nanostructures. [Full article]
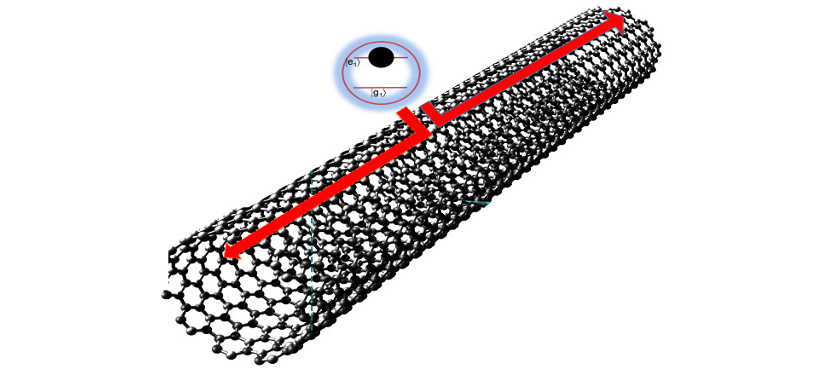
The quest for schemes leading to efficient coupling between quantum emitters and the electromagnetic field is very actively pursued nowadays, for its potential applications to quantum information and to induce effective interactions between two-level systems (mediated by the electromagnetic field) and effective photon-photon interactions (mediated by the quantum emitters).
In this context, IFIMAC researcher Francisco J. Garcia-Vidal, in collaboration with Luis Martin-Moreno (ICMA) and Javier Garcia de Abajo (ICFO) have shown that the tunable plasmons of a highly doped single-wall carbon nanotube are a very promising platform for efficient coupling between quantum emitters and the confined plasmon field. Plasmons in these quasi-one-dimensional carbon structures exhibit very deep subwavelength confinement that pushes the coupling efficiency close to 100% over a very broad spectral range. This phenomenon takes place for distances and tube diameters comprising the nanometer and micrometer scales. As opposite to other metallic systems and also to graphene, carbon nanotubes present the crucial advantage of not requiring a spacer to avoid quenching (i.e., irreversible dissipation leading to heating of the metal) of quantum emitters at small separations. This finding not only holds great potential for waveguide Quantum Electrodynamics, in which an efficient interaction between emitters and electromagnetic modes is pivotal, but it also provides a way of realizing quantum strong coupling between several emitters mediated by carbon nanotube plasmons, which can be controlled through the large electro-optical tunability of these excitations. [Full article]
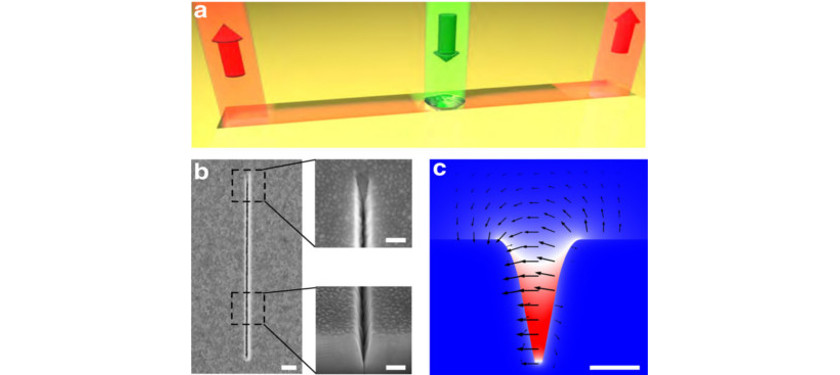
One of the main challenges in developing future nanoscale quantum photonic circuits is to manage combining on a single chip a single photon source, waveguides, modulators and detectors. An important milestone towards this ultimate goal is the deterministic coupling of a single quantum emitter to an integrated waveguide. In this context, IFIMAC researchers Carlos González Ballestero and Esteban Moreno lead by Prof. Francisco J. García Vidal, in collaboration with scientists from ICFO in Barcelona (Romain Quidant´s group), Denmark (Sergey Bozhevolnyi’s group) and United Kingdom (Yuri Alaverdyan), have been able to demonstrate coupling of the emission of a single quantum emitter to channel plasmons polaritons (CPPs) in a V-shaped plasmonic architecture. The study “Coupling of individual quantum emitters to channel plasmons”, has been published recently in the journal Nature Communications.
In this work, the researchers first used theoretical simulations to study the behaviour of the coupling between a quantum emitter and the V-groove plasmonic channel. Once an optimal theoretical configuration was identified, they used state-of-the-art techniques to assemble the experiment using a single Nitrogen Vacancy (NV) centre, a single quantum emitter present in diamond, coupled to the CPPs supported by a V-groove (VG) channel. The observations obtained from the experiment revealed efficient coupling of the NV centre emission to the propagating modes of the VG, in accordance with the theoretical predictions postulated by the team. This demonstrates that their approach can enable realistic and functional plasmonic circuitry and therefore, paves the way towards the development of efficient and long distance transfer of energy in integrated solid-state quantum systems. [Full article]
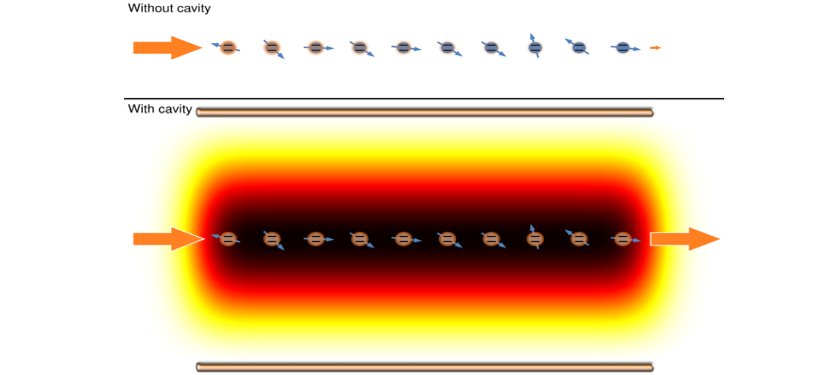
When light is absorbed in organic materials, its energy is converted into excitons (electronic excitations) that normally move around by “hopping” from molecule to molecule. This is important in photosynthesis or solar cells, for example. There, the energy of the absorbed sunlight has to be brought to a “reaction center”, where it can be used to power chemical or electrical processes.
In many organic materials, energy transport is inefficient because of the large amount of disorder and motion of the molecules. The theoretical work, carried out by IFIMAC researchers Johannes Feist and Francisco J. Garcia-Vidal, shows that an extraordinary increase of the transportation efficiency (or “exciton conductance”) can be achieved by placing two mirrors on both sides of the organic material. The excitons can then not only hop from molecule to molecule, but also transfer their energy back into light trapped between the mirrors. When this happens quickly enough, the exciton and light behave as an effective new particle with hybrid properties borrowed from both constituents. In this regime of “strong coupling”, energy transferred into the system at one spot can efficiently “jump” over a large distance, bypassing the disordered collection of molecules. [Full article]
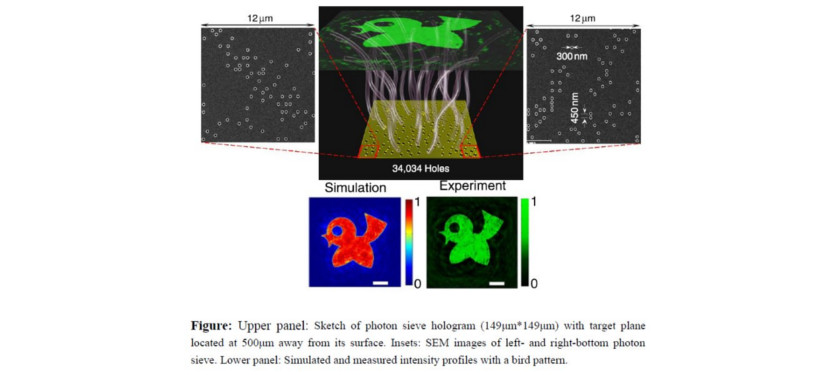
Nano-scale manipulation of light is an important topic in Nanophotonics. Many nanoscale structures are used to control the polarization, intensity, phase and wavelength of light. However, both the difficulty in designing high-capacity photonic devices with extremely rich nanostructures, and the lack of reliable numerical methods for their modeling severely refrain the development of real-world nanophotonic devices. We know that if we had a powerful numerical tool to design devices possessing a large number of fine/rich nanoscale features, we would be able to reach a new flatland with unprecedented opportunities.
Thanks to a joint theory/experiment collaborative project between the director of IFIMAC, Prof. Francisco J. Garcia-Vidal, and two experimental groups based in Singapore, an important leap forward into this direction has been recently achieved. These researchers have been able to design and test two types of nanosieves, both composed of thousands of nanoholes, devised to realize two different functionalities: i) a high-quality hologram and ii) a sub-diffraction spot in the far field. The nanosieve hologram is composed of 34034 nanoholes that are randomly distributed and whose sizes are tuned. The device shows good uniformity, high diffraction efficiency and its performance is polarization-independent. Also, as a difference with previous approaches, it does not produce twin images, which could lead to benefits across fundamental physics research and industrial applications. Using a completely different design, these researchers have also devised a nanosieve that is able to generate a sub-diffraction-limit focusing spot of size 0.32 λ well below the diffraction limit, emerging in the far field and in air ambient conditions. This new nanosieve will have profound implications in the research field of high-resolution imaging and focusing. [Full article]
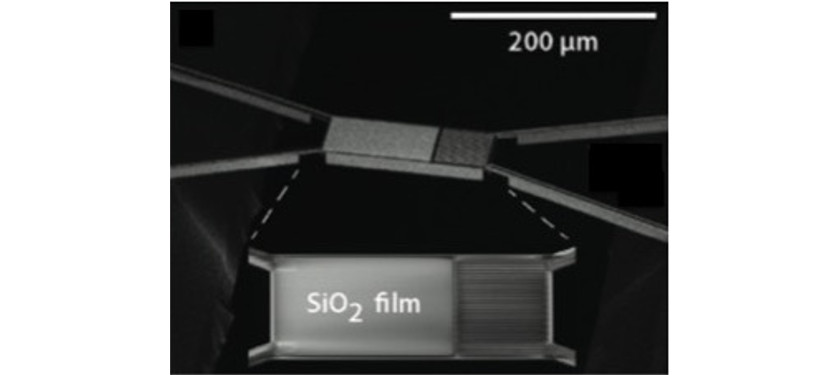
Thermal radiation plays a major role in energy conversion, thermal management, and data storage. In recent years, several experiments on thermal radiation between bulk materials have demonstrated that radiative heat transfer can be greatly enhanced in nanoscale gaps. However, it was not clear whether such enhancements could be obtained with nanoscale films thinner than the penetration depth of radiation. In this work, our colleagues of the University of Michigan (the groups of Pramod Reddy and Edgar Meyhofer) have conducted near-field radiation experiments using a novel ultrasensitive calorimeter that demonstrate enhancements of several orders of magnitude in radiative heat transfer, even for ultra thin dielectric films (50 nm), at spatial separations comparable to or smaller than the film thickness. Researchers from IFIMAC (V. Fernández-Hurtado, J. Feist, F. J. García-Vidal and J. C. Cuevas) have explained these striking results making use of the theory of fluctuational electrodynamics. In particular, we have showed that the near field radiative heat transfer in polar dielectric thin films is determined by the excitation of cavity surface phonon polaritons. These surface electromagnetic modes have characteristic penetration depths that are of the order of the gap separating the receiver from the emitter. In practice, this implies that the entire near field thermal radiation emitted by a polar material comes from its surface. Thus, the thermal emission of a polar thin film is independent of its thickness, as long as the gap between materials remains smaller than the film thickness. Our findings have important implications to a variety of future energy conversion and heat transfer nanotechnologies. [Full article]